The Center for Gravitational Experiments (CGE) has been engaged in the research on Gravitational Physics and related fields, precision gravity measurement, and precision measurement based on Atomic, Molecular and Optical Physics since 1983. It is now the only research center in China that is involved in gravitational experiments, and is a well-known research center for the precision measurement of the Newtonian gravitational constant G. In recent years, numerous novel precision measurement techniques have been developed in the center, e.g. ultra-weak force detection and calibration based on the torsion pendulum technique, capacitive displacement sensing, fiber-optic displacement sensing, precision measurement of gravitational acceleration based on atom interferometry, cryogenic scanning probe microscopy, precision heterodyne laser interferometry, etc. The related research fields compose of Precision Measurement Physics, Atomic and Molecular physics, Theoretical Physics, Radio Physics, Optics and Geophysics.
The CGE currently has six research groups with 69 permanent staffs. there are more than 30 Post-Doc fellows and 100 PhD students working in the center.
(1) Research subjects
The CGE has been involved in the research of Precision Measurement for more than thirty years. Currently there are three main research directions:
A) Gravitational Physics and related fields
The four known fundamental forces in nature, gravity, electromagnetic interaction, weak, and strong interactions, are described by general relativity and the standard model, respectively. However, these two theories appear to be fundamentally incompatible. To connect gravity to the rest of physics, two of the most seriousproblems still exist: the hierarchy problem and the cosmological constant problem. Based on the ideas of string theory or M theory, a number of speculations have been proposed and predict some new physics phenomenal, such as the deviation from the gravitational inverse-square law (ISL) in a short-range regime, the violation of the weak equivalence principle (WEP), and the variation of fundamental constants (G and α, etc.). For the G measurements, two different kinds of experiments are performed, one with the time-of-swing method and another with angular-acceleration-feedback method, with an uncertainty goal of 15 ppm, and try to identify the systematic errors that have not been found previously in the two methods. For the experimental test of the Newton‟s inverse-square law, the group are trying to increase the test precision to explore the parameter space of new physics, such as the physics of large extra dimensions and the axion-like particle mediated interactions, and so on. We will also perform the test for the spin-dependent interactions that are proposed in theories beyond the standard model of particle physics. For the test of the equivalence principle, our goal is to measure the Eötvös parameter with an uncertainty of 10-14~10-15, and check the foundation of the general relativity at higher precision. The groupis also designing experiment for measuring the vacuum magnetic birefringence, which may give direct experimental confirmation on the very fundamental QED prediction. Furthermore, the group will also take part in the TIANQIN project, a Chinese mission for gravitational wave detection in space. In addition, the group will develop a new research direction studying the low dimensional quantum physics, for example quantum Hall effect and low dimensional superconductive, especially their application in quantum precision measurement.
B) Precision gravity measurement
The research direction includes development of space electrostatic accelerometers (SEA), MEMS accelerometer gravity gradiometer (MAG), and superconducting gravimeter and gradiometer (SGG). The main research direction of the SEA group is the development and application of the precision inertial sensor. This kind of sensors have ultra-high sensitivity and low noise, and are typically used in space missions, such as gravitational wave detection, satellite Earth‟s gravity recovery missions, and space fundamental physics test missions. The MAG group focus on the rotating-accelerometers-based gravity gradiometers. Gravity gradient measurements in the moving-base environment are extremely challenging as they aim at extracting Scientific Research 40 minute variations of the gravitational acceleration from the background noise that is many orders of magnitude larger than the signals. Researches are performed on ultra-high-sensitivity accelerometer, long-term dimensional stability, high material tolerances, mismatch factors along with vibration isolation, angular stabilization, read-out techniques, and advanced signal processing. The SGG group focuses on the development of superconducting gravity measurement instruments, including superconducting gravimeter and gravity gradiometer. By incorporating superconductivities and cryogenic technologies into the design, the group aims to construct advanced instruments to improve the resolution of relative gravity measurement, and thus promote the technological level for geophysical observation and geodetic surveys. Compared with the gravity measurement, the moving-based gravity gradient exploration has a number of advantages, including high spatial resolution and precision field source description in inertial navigation applications, geodetic research, unmanned aerial vehicles, and natural resource exploration. Aiming at moving-platform-based (aircraft, ship, submarine, etc.) MEMS rotating accelerometer gravity gradiometer development, the group will develop the fundamental technologies including the stability of the high-sensitivity silicon-based force-balance flexure accelerometer, the matching of the sensitivities of several accelerometers, the airborne gravity gradiometer engineering prototype, and the system reliability in aeronautical environment. We expect to make breakthroughs on the key technologies including the development and reliability optimization of the silicon-based accelerometer with high stability and high sensitivity, the modular design of the dynamic sensitivity-matching of several accelerometers, the integration technology of the gravity gradient sensor with the air-borne platform and the environmental adaptability test technologies of the gravity gradiometer systems engineering prototype. This instrument will support the gravity resources exploration and navigation application.
C) Precision measurement based on Atomic, Molecular and Optical Physics
This direction includes cold atom interferometry for g measurement (AIM) and time and frequency standards (TFS). AIM group started the research of cold atom interferometry in 2006. On the one hand, the AIM group are developing 10-10 g level atom gravimeters in resolution and 10-9 g level in accuracy, which will serve as gravity benchmark for characterizing other gravimeters and geophysics research. On the other hand, compact atomic gravimeters with a precision of several 10-8 g for field applications are also under development. Gravity gradient measurement is also very important. Important applications have been found in scientific and technical areas, ranging from measurements of the gravitational constant and tests of general relativity to the gravity gradient survey. We have proposed an atom interferometry based scheme to determine the “Big G”, in which a dual 3D magneto-optical trap (MOT) configuration and a 1D optical lattice technique are combined to generate high quality atomic fountains and to lower the quantum projection noise. The goal for the planned “Big G” measurement uncertainty is 100 ppm. To achieve this, we are building a high resolution atomic gravity gradiometer with a sensitivity of 60 E/√Hz and a baseline of 0.3 m. With an integration time of 100 hours, the theoretical resolution of G value can reach 38 ppm. We will further improve the test precision of spin related WEP by exploiting internal state invariant atom interferometers (such as Bragg interferometer or Raman double diffraction interferometer) or by constructing a more homogeneous bias magnetic field as quantization axis.
Moreover, atoms in different spin state (hyperfine level) will be explored to test possible new interactions. Searching for new breaking mechanism of the EP is another important development trend. We focus on whether the intrinsic spin of atom is coupled with gravity or space-time torsion, and therefore leads to the breaking of the EP. New atom interferometers employing Bragg diffractions will be constructed to test EP at 10-10 level with atoms in different internal states. In addition, another fundamental hypothesis of general relativity, Lorenz invariance, will be tested using atom interferometry at unprecedented precision in the near future. Molecules are increasingly important for testing fundamental physics since they have rich internal energy level structure. We intend to expand a cold molecular platform for electron electric dipole moment measurement (EDM), aiming at the best accuracy of the world. Laser cooling molecules and a molecular fountain will be implemented.
TFS group started the research on optical frequency standards in 2010, with goals of developing high precision optical and microwave clocks and related techniques for fundamental physical laws test. TFS is currently working on Mg+ /Al+ ion optical frequency standard based on quantum logic technique, narrow line width lasers based on ultra-stable Fabry-Perot cavities and whispering gallery mode cavities, Cs fountain Scientific Research 42 clock, cryogenic sapphire oscillators (CSO), laser gyroscopes, and time and frequency distribution. Research progress including trapping of a single 25Mg+ ion in a linear Paul trap and cooled to the vibrational ground state through Raman sideband cooling technique. The frequency of the 25Mg+ ion ground state hyperfine transition between |S1/2, F = 2, m = 0> and |S1/2, F = 3, m = 0> is measured to 4.2 Hz uncertainty level, which is more than one order of magnitude better than that of NIST‟s result. A single 25Mg+ ion and a single 27Al+ ion are loaded efficiently through laser ablation in a linear Paul trap. The 25Mg+ - 27Al+ ion pair is sympathetic sideband cooled and about 80% population is at ground state. The frequency instability of the ultra-stable lasers based on a 10-cm-long reference cavity has achieved a level of 4.9×10-16, which is limited by the thermal noise of the cavity. Currently it is the best result achieved among all similarly designed 10-cm long all-ULE cavities. The frequency instability of the ultra-stable laser based on a 30-cm-long reference cavity is 3×10-16 at 3-5 s. We are building large scale passive laser gyroscopes with ultra-stable lasers as the light sources. A 9×10-8 rad/s/√Hz rotation measurement sensitivity is achieved with a 1 m×1 m gyroscope. A CSO has been built with a frequency stability of 10-12 at 1 s, limited by the measurement device. We are building a second system to perform a direct comparison. To help carrying out the research, a time and frequency research platform has been set up with equipments including Hydrogen maser, Cs beam clock, femtosecond frequency combs, frequency quadruple UV lasers, pulse tube cryostats, and many high-end electronics devices, such as spectrum analyzers, wave-meters, phase noise analyzers, network analyzers, etc. In the future, new optical frequency standard ideas will be pursued in TFS group, including direct laser cooled Al+ ion optical frequency standard with better stability and accuracy. Taking advantage of the synergy of different frequency standards under development in the group, the research goal is to (a) test fundamental physical laws, including Local Lorentz Invariance, Local Position Invariance, Lense-Thirring effect, etc.; (b) measure fundamental constants and their time variance with better accuracy; (c) perform relativistic geodesy; (d) develop frequency standards in space for gravitational wave detection and testing of general relativity; (e) research quantum metrology technique to further improve the current detection limit.
(2) Group Members
Founder : Professor Luo Jun
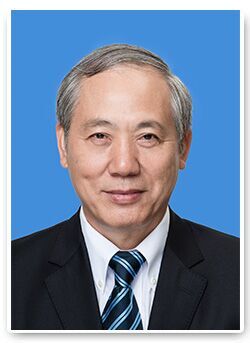
Professor Jun Luo is honored with the academician of the Chinese Academy of Science. He engages in gravitational experiments since 1983. His major achievements in science include:
Using the time-of-swing method to measure the precision value of the Newtonian gravitational constant, and the measured value had been recorded by CODATA;
Using macroscopic rotating objects to test the Equivalence Principle of General Relativity;
Using the modulated torsion balance to determine the upper limit of the photon rest mass, and the result had been recorded by the Particle Data Group;
Using the precision torsion pendulum with dual-frequency modulation to test the Newtonian inverse-square law at the sub-millimeter range.
Other members:
Lu Zehuang, Yang Shanqing, Hu Zhongkun, Liu xiangdong, Pan Minghu, Luo Pengshun, Shao Chenggang, Tu Liangcheng, Wu Shuchao, Xu Zhifang, Ye Xianji, Zhou Zebing, Chen Liang, Jean-MIchel Guy Le FLoch, Liu Jinquan, Wang Yan, Zhang Jie, Zhou Minkang, Lin Guoping, Cao Lushuai, Wang Shun, Fan Ji, Bai Yanzheng, Duan Xiaochun, Deng Ke, Qu Shaobo
3) Research Facilities
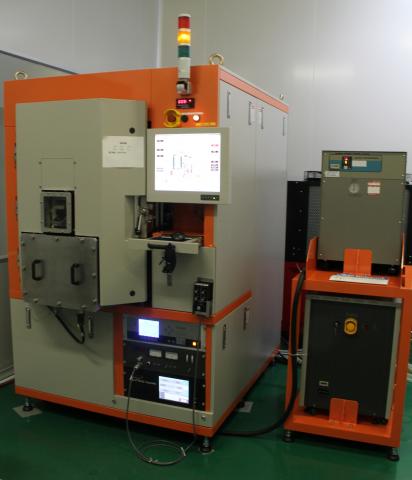
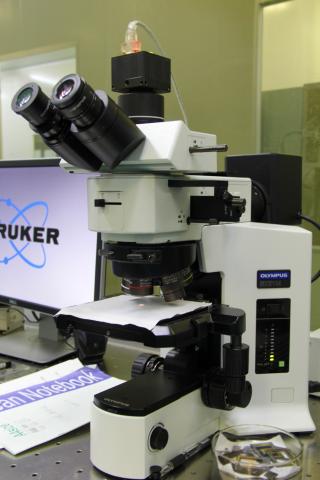
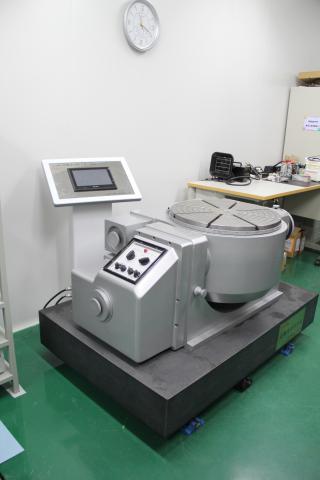
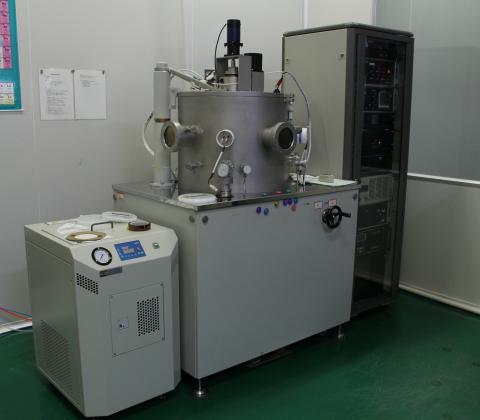